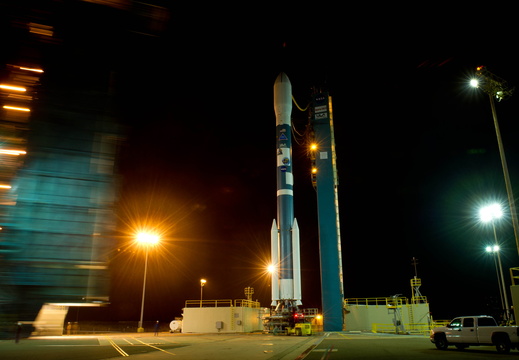
Aquarius/SAC-D
Aquarius/SAC-D mapped the salinity (the concentration of dissolved salt) at the ocean surface, information critical to improving our understanding of two major components of Earth's climate system: the water cycle and ocean circulation. By measuring ocean salinity from space, Aquarius provided new insights into how the massive natural exchange of freshwater between the ocean, atmosphere and sea ice influences ocean circulation, weather and climate.
Because ocean surface salinity varies from place to place and over time, scientists can use it to trace the ocean's role in Earth's water cycle. For example, more than 85 percent of global evaporation and more than 75 percent of global precipitation occur over the ocean. By measuring changes in ocean surface salinity caused by these processes, as well as by ice melting and river runoff, Aquarius/SAC-D provided important new information about how Earth's freshwater moves between the ocean and atmosphere and around the globe.
Knowing ocean surface salinity can also help scientists track ocean currents and better understand ocean circulation. Salinity, together with temperature, determines how dense and buoyant seawater is. This, in turn, drives how ocean waters are layered and mixed and the formation of water masses. Salinity also has a major effect on ocean circulation, including the flow of currents that move heat from the tropics to the poles.
Aquarius/SAC-D provided essential ocean surface salinity data needed to link the water cycle and ocean circulation-two major components of the climate system. This information, in turn, will help scientists improve the accuracy of computer climate models.
Global ocean salinity has been an area of much scientific uncertainty. Past measurements of salinity have been limited mostly to summertime observations in shipping lanes. Recently, a European mission has begun making ocean surface salinity measurements. With the launch of Aquarius/SAC-D, scientists were able to collect more data in the mission's first few months than had been amassed by ships and in-water sensors during the previous 125 years.
Aquarius/SAC-D was designed to measure ocean surface salinity for at least three years, repeating its global pattern every seven days. During its lifetime, the mission provided monthly maps of global changes in ocean surface salinity with a resolution of 150 kilometers (93 miles), showing how salinity changes from month-to-month, season-to-season and year-to-year. The spacecraft flew in a sun-synchronous orbit 657 kilometers (408 miles) above Earth's surface.
NASA's Aquarius was the primary instrument on the SAC-D spacecraft. It consisted of three passive microwave radiometers to detect the surface emission that was used to obtain salinity and an active scatterometer to measure the ocean waves that affected the precision of the salinity measurement. While salinity levels in the open ocean generally range from 32 to 37 practical salinity units, or psu (roughly equivalent to parts per thousand), the Aquarius sensor was able to detect changes in salinity as small as 0.2 psu. This is equivalent to about a "pinch" (i.e., 1/8 of a teaspoon) of salt in one gallon of water.
Aquarius/SAC-D was a collaboration between NASA and Argentina's space agency, Comision Nacional de Actividades Espaciales (CONAE), with participation from Brazil, Canada, France and Italy. The Aquarius instrument was jointly built by NASA's Jet Propulsion Laboratory, Pasadena, California, and NASA's Goddard Space Flight Center, Greenbelt, Maryland. JPL managed Aquarius through the mission's commissioning phase and archived mission data. Goddard managed the mission's operations phase and processed Aquarius science data. NASA's Launch Services Program at the Kennedy Space Center in Florida is managing the launch. CONAE provided the SAC-D spacecraft, an optical camera, a thermal camera in collaboration with Canada, a microwave radiometer, sensors developed by various Argentine institutions, and the mission operations center in Argentina. France and Italy also contributed instruments.
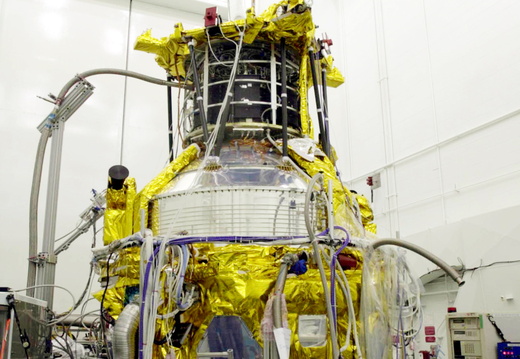
Gravity Probe B
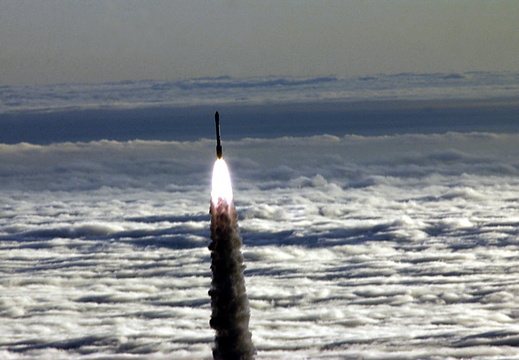
ICESat/CHIPS
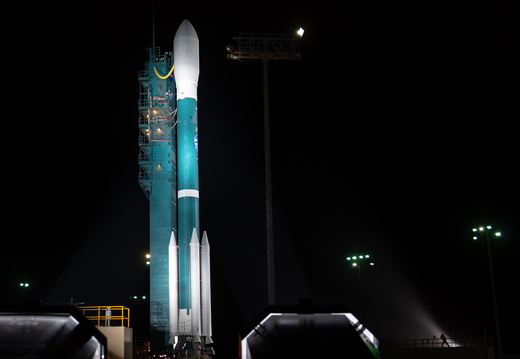
ICESat-2
The Ice, Cloud and land Elevation Satellite-2, or ICESat-2, will measure the height of a changing Earth – one laser pulse at a time, 10,000 laser pulses a second. Launched in 2018, ICESat-2 will carry a laser altimeter that detects individual photons, allowing scientists to measure the elevation of ice sheets, sea ice, forests and more in unprecedented detail.
Our planet's frozen and icy areas, called the cryosphere, are a key focus of NASA's Earth science research. ICESat-2 will help scientists investigate why, and how much, our cryosphere is changing in a warming climate. The satellite will also measure heights across Earth's temperate and tropical regions, and take stock of the vegetation in forests worldwide.
Using Light to Measure Height
ICESat-2 will carry a single instrument, the Advanced Topographic Laser Altimeter System, or ATLAS. ATLAS has a single laser, split into six beams and arranged in three pairs to better gauge the slope of Earth's surface. Mechanisms on board precisely time the round-trip of laser photons as they leave ATLAS, reflect off the ground and return to the receiver telescope. By matching those times with the satellite's precise location in space, mission scientists will be able to determine the heights of Earth’s surface.
With 10,000 laser pulses per second, this fast-shooting laser technology allows ATLAS to take measurements every 28 inches along the satellite's path. The mission will gather enough data to estimate the annual height change of Greenland and Antarctic ice sheets to within 4 millimeters – the width of a No. 2 pencil.
ICESat-2 advances our laser technology from the first ICESat, which was launched in 2003 and operated until 2009. While ICESat-2 will carry ATLAS, the first ICESat carried the Geoscience Laser Altimeter System (GLAS) instrument – the first laser-ranging system that made continuous Earth observations. The single-beam GLAS laser fired 40 pulses a second in infrared light, taking measurements every 170 meters along the satellite's track on Earth's surface. As a comparison, if the two instruments took measurements over a football field, GLAS would have collected data points outside the two end zones, but ICESat-2's ATLAS would take measurements between each yard line.
Science GoalsIn its three-year design lifespan, ICESat-2 will document the ebbs and flows of ice sheets, the shrinking or growth of sea ice, the height of the world's forests and more. When data from ICESat-2 are combined with what scientists have learned from the first ICESat and Operation IceBridge, an ongoing aerial campaign that started in 2009, researchers will be able to track the cryosphere for a 16-year span.
With the new technological advancements that ATLAS will bring, the ICESat-2 mission is designed to accomplish four science objectives:
Measure how much melting ice sheets in Greenland and Antarctica contribute to sea level changes Measure how ice sheets, glaciers and more are gaining or losing mass in different regions, to help researchers understand the mechanisms behind those changes Estimate the thickness of sea ice, and monitor any changes Measure the height of forests to calculate the amount of vegetation in a region
KEPLER
The centuries-old quest for other worlds like our Earth has been rejuvenated by the intense excitement and popular interest surrounding the discovery of hundreds of planets orbiting other stars. There is now clear evidence for substantial numbers of three types of exoplanets; gas giants, hot-super-Earths in short period orbits, and ice giants. The challenge now is to find terrestrial planets (i.e., those one half to twice the size of the Earth), especially those in the habitable zone of their stars where liquid water might exist on the surface of the planet.
The Kepler Mission is specifically designed to survey our region of the Milky Way galaxy to discover hundreds of Earth-size and smaller planets in or near the habitable zone and determine the fraction of the hundreds of billions of stars in our galaxy that might have such planets.
Kepler ScienceThe scientific objective of the Kepler Mission is to explore the structure and diversity of planetary systems. This is achieved by surveying a large sample of stars to:
Determine the percentage of terrestrial and larger planets that are in or near the habitable zone of a wide variety of stars Determine the distribution of sizes and shapes of the orbits of these planets Estimate how many planets there are in multiple-star systems Determine the variety of orbit sizes and planet reflectivities, sizes, masses and densities of short-period giant planets Identify additional members of each discovered planetary system using other techniques Determine the properties of those stars that harbor planetary systems. The Transit Method of Detecting Extrasolar PlanetsWhen a planet passes in front of a star as viewed from Earth, the event is called a “transit”. On Earth, we can observe an occasional Venus or Mercury transit. These events are seen as a small black dot creeping across the Sun—Venus or Mercury blocks sunlight as the planet moves between the Sun and us. Kepler finds planets by looking for tiny dips in the brightness of a star when a planet crosses in front of it—we say the planet transits the star.
Once detected, the planet's orbital size can be calculated from the period (how long it takes the planet to orbit once around the star) and the mass of the star using Kepler's Third Law of planetary motion. The size of the planet is found from the depth of the transit (how much the brightness of the star drops) and the size of the star. From the orbital size and the temperature of the star, the planet's characteristic temperature can be calculated. From this the question of whether or not the planet is habitable (not necessarily inhabited) can be answered.
Target Field of ViewSince transits only last a fraction of a day, all the stars must be monitored continuously, that is, their brightnesses must be measured at least once every few hours. The ability to continuously view the stars being monitored dictates that the field of view (FOV) must never be blocked at any time during the year. Therefore, to avoid the Sun the FOV must be out of the ecliptic plane. The secondary requirement is that the FOV have the largest possible number of stars. This leads to the selection of a region in the Cygnus and Lyra constellations of our Galaxy as shown.
Learn more about the history of the mission from the account of Kepler visionary and principal investigator, William Borucki.
Kepler's second mission: K2The loss of a second of the four reaction wheels on board the Kepler spacecraft in May 2013 brought an end to Kepler's four-year science mission to continuously monitor more than 150,000 stars to search for transiting exoplanets. Developed over the months following this failure, the K2 mission represents a new concept for spacecraft operations that enables continued scientific observations with the Kepler space telescope. K2 became fully operational in May 2014 and is expected to continue operating until 2017 or 2018.
Using the transit method to detect brightness changes, the K2 mission entails a series of sequential observing "Campaigns" of fields distributed around the ecliptic plane and offers a photometric precision approaching that of the original Kepler mission. Operating in the ecliptic plane minimizes the torque exerted on the spacecraft by solar wind pressure, reducing pointing drift to the point where spacecraft attitude can effectively be controlled through a combination of thrusters and the two remaining reaction wheels. Each campaign is therefore limited by sun angle constraints to a duration of approximately 80 days.
Scientific motivations
K2 is a community-driven mission. All K2 targets are proposed by the community through the Guest Observer program. The scientific potential spans a wide range of astrophysics. It is anticipated that K2 will:
Provide a yield of hot planets around bright stars for follow-up transit spectroscopy to facilitate rapid advances in the characterization of exoplanet atmospheres. Provide a yield of small planets around bright, small stars to facilitate the most precise follow-up measurements to date of masses, densities and compositions. Identify locations and characteristics of potentially-habitable planets around bright M-dwarfs in the solar neighborhood. Determine if hot gas giants exist around young stars, or whether they migrate to small orbits at a later epoch by tidal or other interactions. Determine the relationship between stellar structure, rotation and activity within stellar associations over a range of ages and metallicity. Identify the progenitors of Type Ia supernovae from photometric structure in the rise to outburst maximum. Discover and characterize binary stars within open clusters and stellar associations. Characterize internal stellar structure and fundamental properties of stars using the tools of asteroseismology. Provide a large, regular cadence survey of AGN activity in the optical bandpass. Participate in multi-mission, multi-band monitoring campaigns of ecliptic targets along with other space-based hardware or ground-based telescopes.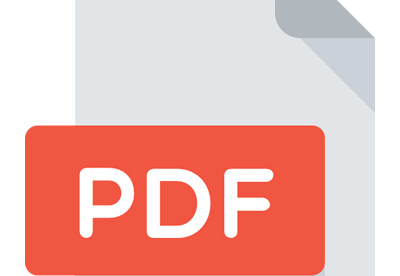
Spitzer
The Spitzer Space Telescope was the final mission in NASA's Great Observatories Program - a family of four space-based observatories, each observing the Universe in a different kind of light. The other missions in the program include the visible-light Hubble Space Telescope (HST), Compton Gamma-Ray Observatory (CGRO), and the Chandra X-Ray Observatory (CXO).
Spitzer was designed to detect infrared radiation, which is primarily heat radiation. It was comprised of two major components:
The Cryogenic Telescope Assembly, which contained the 85 centimeter telescope and Spitzer's three scientific instruments.
The Spacecraft, which controlled the telescope, provided power to the instruments, handled the scientific data and communicated with Earth.
It may seem like a contradiction, but NASA's Spitzer Space Telescope had to be simultaneously warm and cold to function properly. Everything in the Cryogenic Telescope Assembly had to be cooled to only a few degrees above absolute zero (-459 degrees Fahrenheit, or -273 degrees Celsius). This was achieved with an onboard tank of liquid helium, or cryogen. Meanwhile, electronic equipment in The Spacecraft portion needed to operate near room temperature.
Spitzer's highly sensitive instruments allowed scientists to peer into cosmic regions that are hidden from optical telescopes, including dusty stellar nurseries, the centers of galaxies, and newly forming planetary systems. Spitzer's infrared eyes also allowed astronomers to see cooler objects in space, such as failed stars (brown dwarfs), extrasolar planets, giant molecular clouds, and organic molecules that may hold the secret to life on other planets.
Spitzer was originally built to last for a minimum of 2.5 years, but it lasted in the cold phase for over 5.5 years. On May 15, 2009 the coolant was finally depleted and the Spitzer "warm mission" began. Providing imaging with two channels (at 3.6 and 4.5 microns) in one of its instruments (IRAC), Spitzer continued to operate until January 30, 2020. The warm mission became the "Spitzer Beyond" mission in August 2016. NASA announced in May 2019 that it would end the Spitzer mission on January 30, 2020, naming the remaining mission the “Spitzer Final Voyage”.
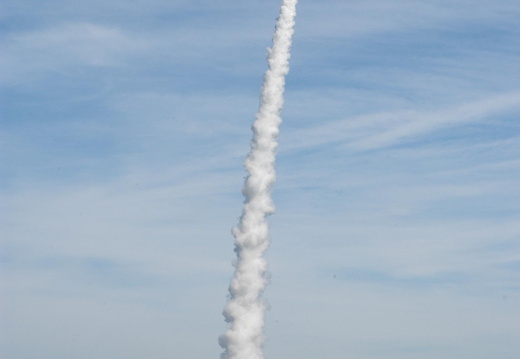
SWIFT
By the end of this day, somewhere in the visible universe a new black hole will have formed. Gamma-ray bursts (GRBs), the most distant and powerful explosions known, are likely the birth cries of these new black holes.
NASA’s Swift mission is dedicated to studying the gamma-ray burst/black hole connection.
“Swift caps off a 30-year hunt to understand the nature of gamma-ray bursts, flashes of light that burn as brightly as a billion billion suns,” said Dr. Anne Kinney, Director of the Universe Division, NASA Headquarters, Washington. “Swift is fine-tuned to quickly loca
te these bursts and study them in several different wavelengths before they disappear forever. Swift is a little satellite with a big appetite,” she said.
Gamma-ray bursts are fleeting events, lasting only a few milliseconds to a few minutes, never to appear in the same spot again. They occur from our vantage point about once a day. Some bursts appear to be from massive star explosions that form black holes.
The Swift observatory comprises three telescopes, which work in tandem to provide rapid identification and multi-wavelength follow-up of GRBs and their afterglows. Within 20 to 75 seconds of a detected GRB, the observatory will rotate autonomously, so the onboard X-ray and optical telescopes can view the burst. The afterglows will be monitored over their durations, and the data will be rapidly released to the public.
The afterglow phenomenon follows the initial gamma-ray flash in most bursts. It can linger in X-ray light, optical light and radio waves for hours to weeks, providing great detail. The crucial link here, however, is having a precise location to direct other telescopes. Swift is the first satellite to provide this capability with both great precision and speed.
“We expect to detect and analyze over 100 gamma-ray bursts a year,” said Dr. Neil Gehrels, Swift’s Principal Investigator at NASA’s Goddard Space Flight Center (GSFC) in Greenbelt, Md. “Swift will lead to a windfall of discovery on these most powerful explosions in the universe.”